An overview of the main venomous animals that contribute to health science. Today and what is yet to come!
Several animals, both terrestrial and marine, have venom. As representatives, we can mention snakes, spiders, scorpions, bees, fish, frogs, caterpillars, anemones, cone snails, and sponges.
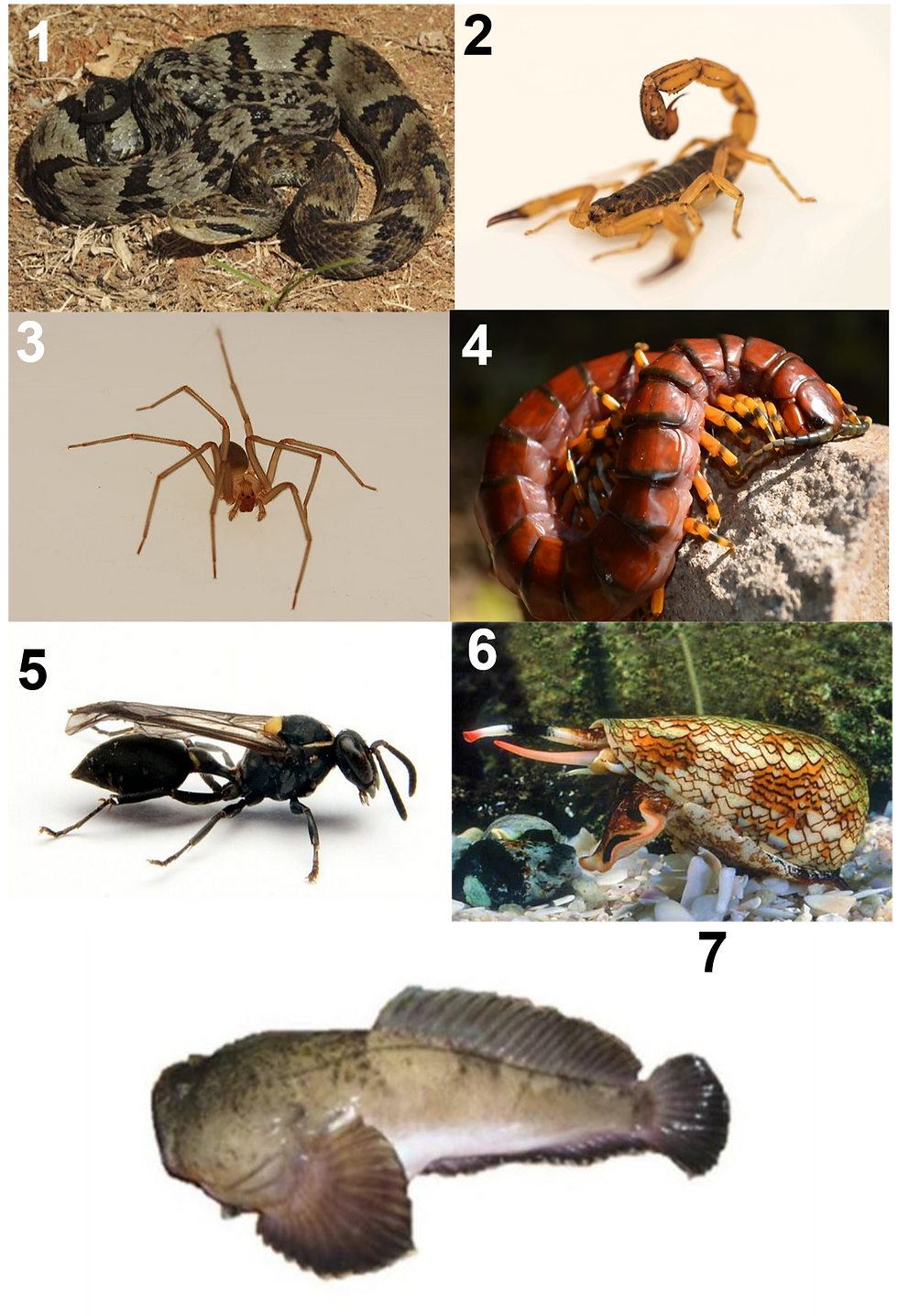
Why do these animals produce venom?
This is just the result of a long evolutionary process and natural selection (remember Darwin?).
Venom's main function is defense, predation, or digestion, but besides their natural or biological activity, our research group, and some others around the world, investigate venoms as sources for the production of medicines.
How come? (!!) Well, to answer this question, follow me in this text, starting with the definition of what are venoms and toxins.
Venoms are mixtures of several molecules called toxins, mainly made up of a variety of enzymes, peptides (the smallest part of a protein), inorganic ions, and nucleotides. They interact with cell receptors, membrane transporters, and ion channels, triggering changes in the body, as for example changes in hemostasis (balance of blood flow), leading to continuous bleeding, or paralysis of some region of the body, neurotoxic action, as shown in the infographic below.
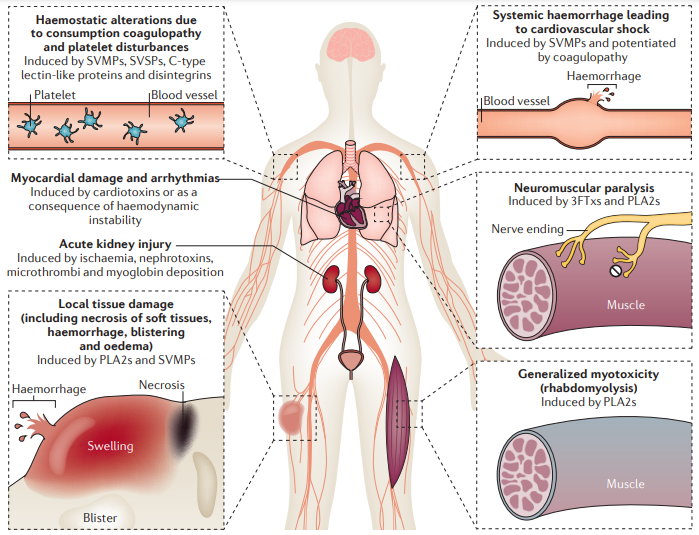
It is estimated that one sample of venom may contain 100 to 500 bioactive components (or toxins). On a larger scale, these numbers could be as high as 10 to 50 million natural compounds available for study, but so far, only 0.01% of this has been explored.
Preclinical studies have shown that venoms and toxins have a great potential for the development of new drugs for the treatment of maladies, such as neurodegenerative, autoimmune diseases, and cancer. Studies generally focus on the four main sources of toxins: snakes, spiders, scorpions, and bees. But other animals are also investigated, as described below.
In neurodegenerative diseases, such as Alzheimer's
Preclinical studies show that snake toxins from the class of dendrotoxins, fasciculins, and metalloproteinases may be allies in the treatment of this disease. The dendrotoxins and fasciculins could help in the release of acetylcholine, a neurotransmitter of great importance for the correct functioning of the brain. Dendrotoxins block ionic channels and fasciculins inhibit the action of the enzyme acetylcholinesterase (responsible for controlling the functioning of acetylcholine), while metalloproteinases can help in the degradation of amyloid-β protein, responsible for the formation of amyloid plaques, characteristic of Alzheimer's. Because of these benefits, these toxins are investigated to better understand their structure and devise derived molecules to help treat the disease.
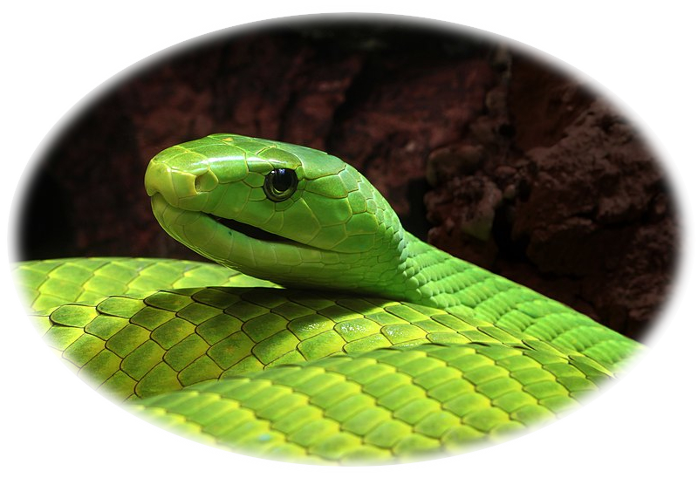
Autoimmune Diseases
Autoimmune diseases are mediated by lymphocytes (the body's defense cells) and result in an abnormal immune response in a certain part of the body under normal conditions. This unusual response is related to a potassium voltage channel, Kv1.3. Studies indicate that the control of this channel would alleviate the exacerbated immune response. Investigations with sea anemone venom have shown promising results, among them, the ShK toxin stands out, due to its specificity, inhibitory activity, and potency in Kv1.3. From this toxin was generated a derivative molecule, the ShK-186, which showed promising results in phase II clinical studies.
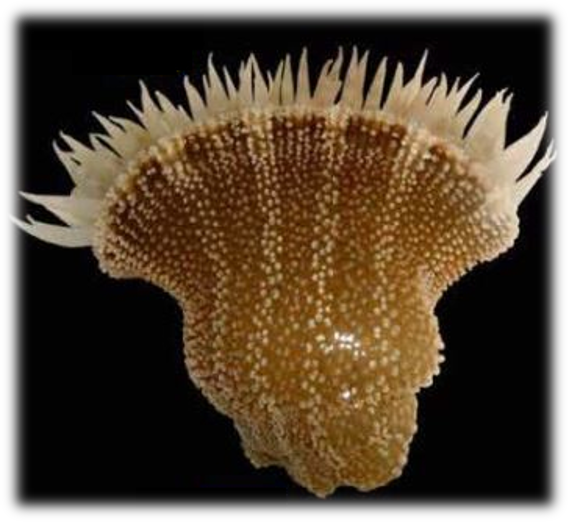
And in cancer?
Snake toxins (metalloproteinases, phospholipases A2, and flavoenzymes) and scorpion toxins (chlorotoxin, iberiotoxin, and charybdotoxin) have shown antitumor action on cells of different tumors such as gliomas (central nervous system), lymphomas (blood), melanomas (skin), and neuroblastoma (neuroendocrine system). They act in different ways, but the final effect observed in these studies is to reduce the ability of the tumor to grow in experimental laboratory models.
But there are also studies in the clinical phase. In one of them, chlorotoxin was associated with a synthetic T-cell receptor that is able to recognize tumor cells, stimulating the immune response against glioblastoma cells.
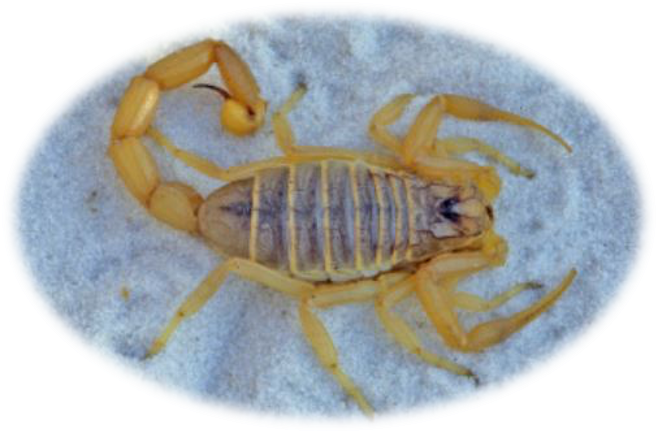
"In our lab we have been studying for more than a decade a toxin from the venom of the Bothrops jararacussu snake, called BJcuL. This toxin showed a toxic action on different tumor cells, at the same time it was shown to be able to activate immune system cells, which would have the potential to help the body fight the tumor. In upcoming posts we will be telling you a little more about our findings."
A recent example. In August 2021, an investigation involving researchers from Brazilian universities (UNESP, USP, UFSCar, and UNIFESP) brought a possible ally in the treatment against Sars-Cov-2, responsible for Covid-19. Derivatives of the toxin bothropstoxin-I, a molecule from B. jararacussu venom, showed a reduction of up to 75% against the virus replication in a monkey cell line. According to the researchers, the next steps involve testing the efficiency of the molecule in different concentrations and if it can promote other actions, such as protecting the cell against virus invasion. After that, it is planned to conduct tests with animals infected with Sars-Cov-2 (Sources, 2021).
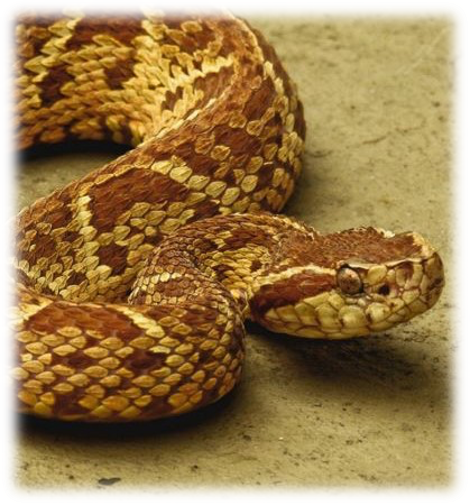
Of the various researches carried out with venoms, some cases have already resulted in the elaboration of drugs, which today are used in the treatment of diseases. Starting with the most classic example, Captopril is the drug that uses a derivative of jararaca venom toxin. Its studies began in the mid-1960s, where the bradykinin potentiating factors were purified and characterized. This group of peptides present in the jararaca venom was related to the decrease in the production of the vasoactive molecule angiotensin, through the inhibition of the angiotensin-converting enzyme, which consequently decreases blood pressure. Later, in the 1980s, a derivative was created from the teprotide toxin, which resulted in the creation of one of the main drugs used in the treatment of hypertension.
Ziconotide (2004) is an analgesic based on one of the toxins in the venom of the marine mollusk Conus magus. This drug has the compound SNX-111, derived from the MVIIA toxin, which acts on Ca2+ ion channels (Cav2.2) and reduces pain transmission along the spinal cord. It is used in cases of chronic pain.

Exenatide (2005) helps in the treatment of type II diabetes and is derived from one of the components of the saliva of the lizard Heloderma suspectum, exandin-4. This drug has a peptide similar to the hormone GLP-1 (glucagon-like peptide-1). Patients with type II diabetes have GLP-1 in low concentrations in their blood during meals, due to its degradation. Exenatide prevents this by interacting with the enzyme that degrades GLP-1, allowing it to last longer in the bloodstream and act on the β-cells of the pancreas. This results in the production of insulin and consequently increases the uptake of glucose by the cells and decreases its concentration in the blood.
"Those were just a few examples of how important research is. It states that the way we view life makes all the difference; a different point of view changes everything!"
The curiosity about animal venoms and toxins has opened a range of knowledge and possibilities that can solve several problems. Within science, there is a multidisciplinary area, responsible for studying the poisons and toxins of animals, plants, and microorganisms. About several aspects, their structure, function, mechanism, origin, evolution, and clinical aspects. This science is called TOXINOLOGY.
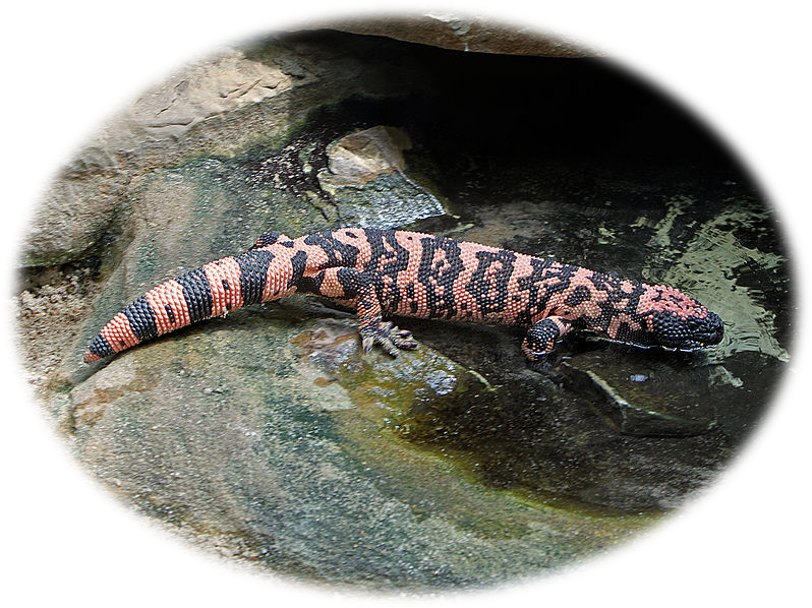
The early studies succeeded in isolating the compounds and depending on the type of toxin, it was needed a large amount of it. With advances in technology, new approaches (proteomics and transcriptomics), and more sensitive methods of molecule detection, different toxins, even at low concentrations, can be selected and characterized without the need for a large sample volume.
"The applications are diverse, going beyond the therapeutic, as described throughout the text. However, the most important is to understand that these animals and their environment need to be preserved, not simply because they are a rich source of toxins with clinical potential. For, like any species, they also have important roles in nature. The loss of one of them creates a gap in both knowledge and function in the environment. This affects various aspects, whether biological, ecological, clinical or therapeutic. "
Sources:
Batista, E. L. (2016). Why scorpions arte now worrisome. Pesquisa Fapesp. https://revistapesquisa.fapesp.br/en/why-scorpions-are-now-more-worrisome/
Bordon, K. de C. F., Cologna, C. T., Fornari-Baldo, E. C., Pinheiro-Júnior, E. L., Cerni, F. A., Amorim, F. G., Anjolette, F. A. P., Cordeiro, F. A., Wiezel, G. A., Cardoso, I. A., Ferreira, I. G., Oliveira, I. S. de, Boldrini-França, J., Pucca, M. B., Baldo, M. A., & Arantes, E. C. (2020). From Animal Poisons and Venoms to Medicines: Achievements, Challenges and Perspectives in Drug Discovery. Frontiers in Pharmacology, 11(July). https://doi.org/10.3389/fphar.2020.01132
Bruni, F. M., Coutinho, E. M. M., Andrade-Barros, A. I., Grund, L. Z., Lopes-Ferreira, M., & Lima, C. (2020). Anaphylaxis induced by Thalassophryne nattereri venom in mice is an IgE/IgG1-mediated, IL-4-dependent phenomenon. Scientific Reports, 10(1), 1–15. https://doi.org/10.1038/s41598-019-57231-y
Carneiro-Goetten, J. O. L., Rodrigues, B. S., Nogoceke, R. A., Do Nascimento, T. G., Moreno-Amaral, A. N., Stuelp-Campelo, P. M., & Elifio-Esposito, S. (2020). Neutrophils activated by BJcuL, a C-type lectin isolated from Bothrops jararacussu venom, decrease the invasion potential of neuroblastoma SK-N-SH cells in vitro. Journal of Venomous Animals and Toxins Including Tropical Diseases, 26(April 2020), 1–10. https://doi.org/10.1590/1678-9199-jvatitd-2019-0073
Chaisakul, J., Hodgson, W. C., Kuruppu, S., & Prasongsook, N. (2016). Effects of animal venoms and toxins on hallmarks of cancer. Journal of Cancer, 7(11), 1571–1578. https://doi.org/10.7150/jca.15309
Chaparro, E., & da Silva, P. I. (2016). Lacrain: the first antimicrobial peptide from the body extract of the Brazilian centipede Scolopendra viridicornis. International Journal of Antimicrobial Agents, 48(3), 277–285. https://doi.org/10.1016/j.ijantimicag.2016.05.015
Chen, N., Xu, S., Zhang, Y., & Wang, F. (2018). Animal protein toxins: origins and therapeutic applications. Biophysics Reports, 4(5), 233–242. https://doi.org/10.1007/s41048-018-0067-x
Citron. (2011). Dendroaspis angusticeps. Wikimedia Commons. https://commons.wikimedia.org/wiki/File:Mamba_Dendroaspis_angusticeps.jpg
Commons, W. (2008). Loxosceles sp. Wikimedia Commons. https://commons.wikimedia.org/w/index.php?search=Loxosceles&title=Special:MediaSearch&go=Go&type=image&haslicense=unrestricted
Coulter-Parkhill, A., McClean, S., Gault, V. A., & Irwin, N. (2021). Therapeutic Potential of Peptides Derived from Animal Venoms: Current Views and Emerging Drugs for Diabetes. Clinical Medicine Insights: Endocrinology and Diabetes, 14(Table 1). https://doi.org/10.1177/11795514211006071
da Silva Aguiar, W., da Costa Galizio, N., Sant’Anna, S. S., Silveira, G. P. M., de Souza Rodrigues, F., Grego, K. F., de Morais-Zani, K., & Tanaka-Azevedo, A. M. (2020). Ontogenetic study of Bothrops jararacussu venom composition reveals distinct profiles. Toxicon, 186(July), 67–77. https://doi.org/10.1016/j.toxicon.2020.07.030
de Souza, J. M., Goncalves, B. D. C., Gomez, M. V., Vieira, L. B., & Ribeiro, F. M. (2018). Animal toxins as therapeutic tools to treat neurodegenerative diseases. Frontiers in Pharmacology, 9(FEB), 1–25. https://doi.org/10.3389/fphar.2018.00145
Diez, Y. L., & Campos-Castro, A. (2016). Soft corals (Anthozoa: Corallimorpharia, Actinaria and Zoantharia) from southeastern of Cuba, and its distribution in Marine Protected Areas. Revista Investigaciones Marinas, 36(May 2017), 80–93. https://www.oceandocs.org/handle/1834/9860
El-Aziz, T. M. A., Soares, A. G., & Stockand, J. D. (2019). Snake venoms in drug discovery: Valuable therapeutic tools for life saving. Toxins, 11(10), 1–25. https://doi.org/10.3390/toxins11100564
Farias, I. B. de, Morais-Zani, K. de, Serino-Silva, C., Sant’Anna, S. S., Rocha, M. M. T. d., Grego, K. F., Andrade-Silva, D., Serrano, S. M. T., & Tanaka-Azevedo, A. M. (2018). Functional and proteomic comparison of Bothrops jararaca venom from captive specimens and the Brazilian Bothropic Reference Venom. Journal of Proteomics, 174(December 2017), 36–46. https://doi.org/10.1016/j.jprot.2017.12.008
Fontes, H. (2021). Veneno de cobra brasileira tem molécula que inibe o coronavírus. Unesp. https://www.iq.unesp.br/#!/noticia/762/veneno-de-cobra-brasileira-tem-molecula-que-inibe-o-coronavirus
Freire, M. C. L. C., Noske, G. D., Bitencourt, V., Sanches, P. R. S., Santos-filho, N. A., Gawriljuk, V. O., Souza, E. P. De, Nogueira, V. H. R., Godoy, M. O. De, Nakamura, A. M., Fernandes, R. S., Godoy, A. S., Juliano, M. A., Peres, B. M., Barbosa, G., Moraes, C. B., Freitas-junior, L. H. G., Cilli, E. M., Guido, R. V. C., & Oliva, G. (2021). Non-Toxic Dimeric Peptides Derived from the Bothropstoxin-I Are Potent SARS-CoV-2 and Papain-like Protease Inhibitors. 2, 1–17.
Gutiérrez, J. M., Calvete, J. J., Habib, A. G., Harrison, R. A., Williams, D. J., & Warrell, D. A. (2017). Snakebite envenoming. Nature Reviews. Disease Primers, 3, 17063. https://doi.org/10.1038/nrdp.2017.63
Harvey, A. L. (2014). Toxins and drug discovery. Toxicon, 92, 193–200. https://doi.org/10.1016/j.toxicon.2014.10.020
Kini, R. M. (2020). Toxinology provides multidirectional and multidimensional opportunities: A personal perspective. Toxicon: X, 6, 100039. https://doi.org/10.1016/j.toxcx.2020.100039
Mullen, G. R., & David Sissom, W. (2019). Chapter 23 - Scorpions (Scorpiones). In G. R. Mullen & L. A. Durden (Eds.), Medical and Veterinary Entomology (Third Edition) (3rd ed., pp. 489–504). Academic Press. https://doi.org/https://doi.org/10.1016/C2017-0-00210-0
Perez-Riverol, A., Fernandes, L. G. R., Musacchio Lasa, A., dos Santos-Pinto, J. R. A., Moitinho Abram, D., Izuka Moraes, G. H., Jabs, F., Miehe, M., Seismman, H., Palma, M. S., de Lima Zollner, R., Spillner, E., & Brochetto-Braga, M. R. (2018). Phospholipase A1-based cross-reactivity among venoms of clinically relevant Hymenoptera from Neotropical and temperate regions. Molecular Immunology, 93(November 2017), 87–93. https://doi.org/10.1016/j.molimm.2017.11.007
Robinson, S. D., Undheim, E. A. B., Ueberheide, B., & King, G. F. (2017). Venom peptides as therapeutics: advances, challenges and the future of venom-peptide discovery. Expert Review of Proteomics, 14(10), 931–939. https://doi.org/10.1080/14789450.2017.1377613
Santos, S. (2021). Veneno de caracol marinho pode ser usado como analgésico a partir da Nanociência. NanoEach. http://www.each.usp.br/nanoeach/?p=2181
Shen, B., Cao, Z., Li, W., Sabatier, J. M., & Wu, Y. (2017). Treating autoimmune disorders with venom-derived peptides. Expert Opinion on Biological Therapy, 17(9), 1065–1075. https://doi.org/10.1080/14712598.2017.1346606
Zell, H. (2011). Heloderma suspectum. Wikimedia Commons. https://commons.wikimedia.org/wiki/File:Heloderma_suspectum_01.jpg
Comments